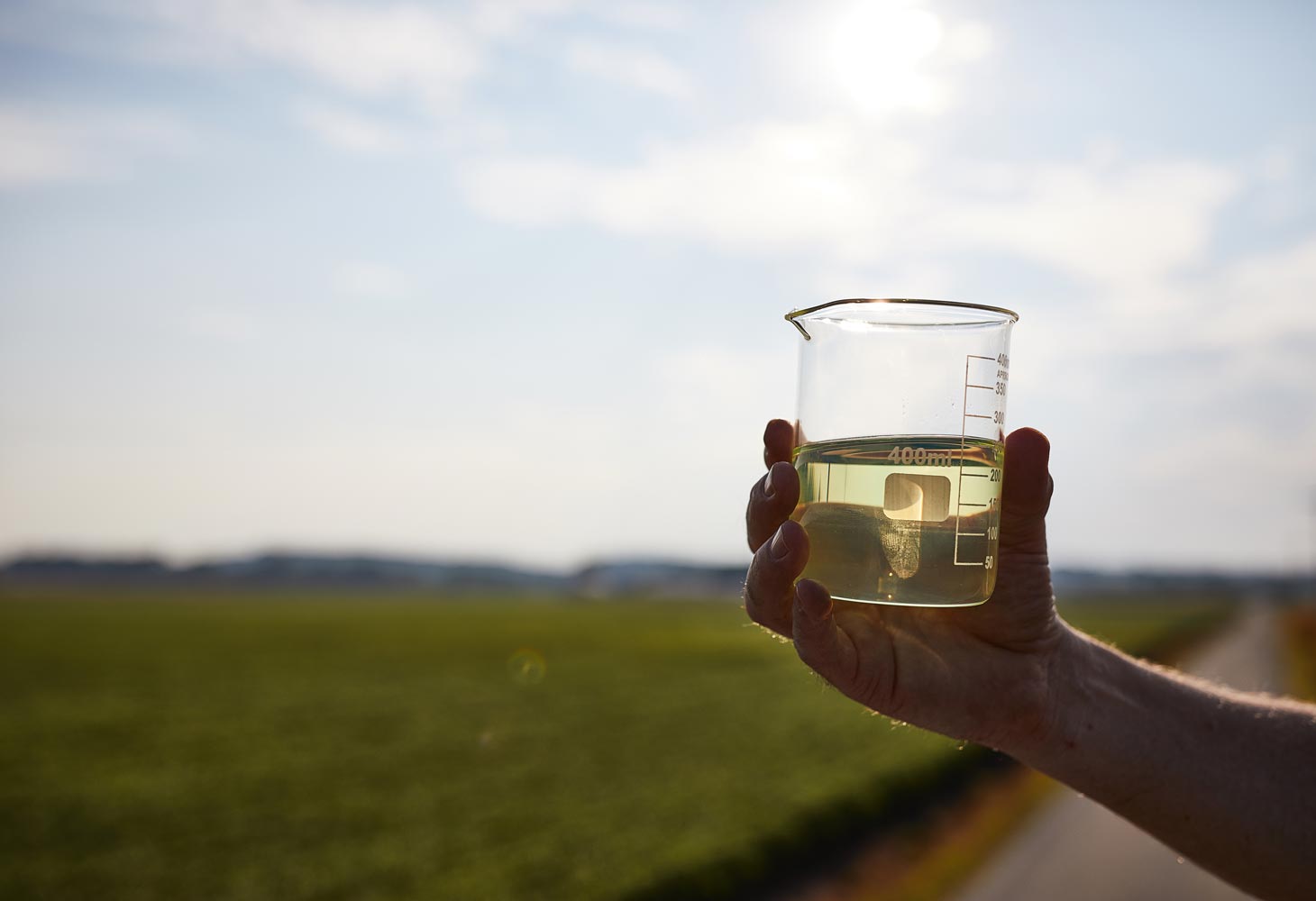
Additives research aiding adoption of EALs for mainstream use
By Raj Shah1, Brajendra K. Sharma2, and Blerim Gashi1
Research on environmentally acceptable lubricants or EALs is growing in popularity as non-traditional parameters centered around sustainability rise in importance within the field of tribological research.
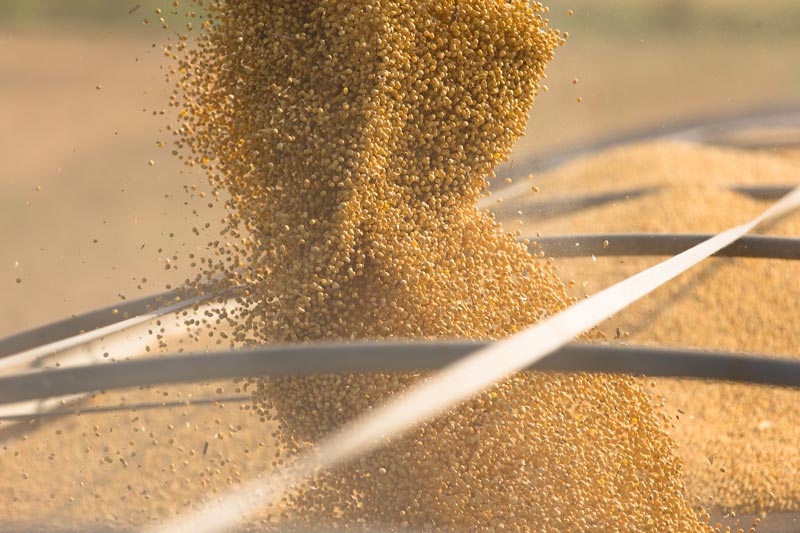
The concept of sustainability widely encompasses environmentally friendly properties such as biodegradation, toxicity, and bioaccumulation while also considering long-term durability and repercussions on human food cycles and deforestation.[1] Biodegradability refers to the persistence of leaked oil in the environment and is commonly assessed using the OECD 301 A/B/C/D/F tests, while toxicity specifically relates to the impact of lubricants on surrounding life, water, or nutrients which is evaluated using the OECD 201-203 and OECD 209-211 tests.[2] Formulations of EALs or bio-lubricants must provide equal or better lubrication and anti-wear properties, at a lower cost to compete with synthetic or petroleum-based formulation. Lubricant engineering thus primarily focuses on the combination of an eco-friendly base oil with exceptional tribological properties and a polymer or nanoparticle additive to bolster both lubrication and condition applicability or range.
For a lubricant to be considered environmentally acceptable, the base oil must have some aspect of renewability, such as being derived from vegetable or animal products. A base oil derived from these sources, such as waste cooking oils (WCOs) and vegetable oils, simultaneously fulfills sustainability requirements. For comparison, mineral oil is a loose term describing a lubricant composed of various petroleum derivatives, in which the most common chemical structures present include paraffinic, aromatic, and naphthenic making mineral oils non-renewable.[3] On the other hand, synthetic esters remain the most environmentally friendly synthetic option. Despite containing polyol structures that are of petrochemical origins, the presence of fatty acids is of natural origin.[3]
Differences imposed by chemical structure
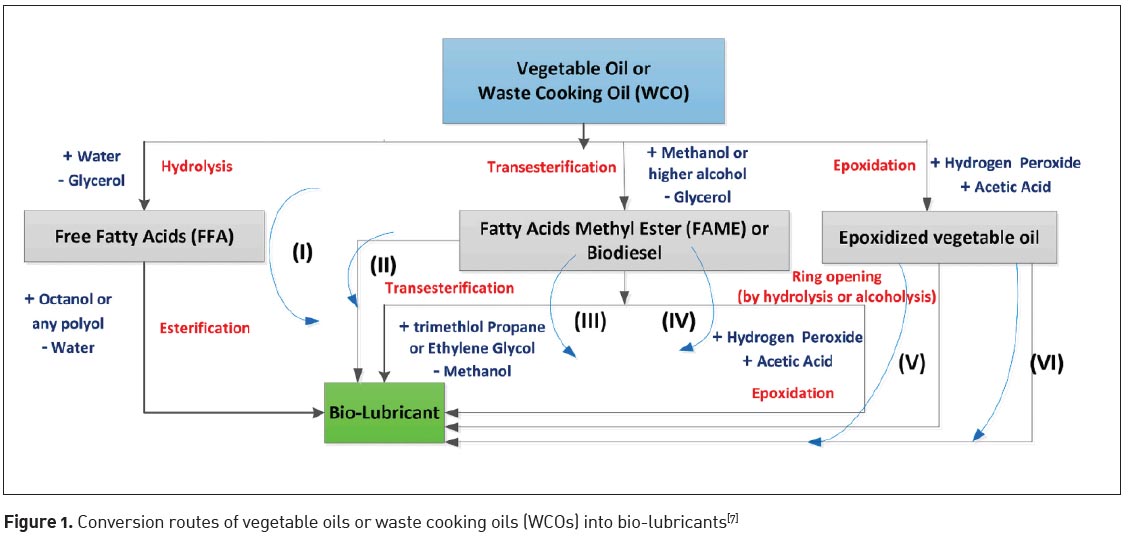
In terms of sustainability, the differences imposed by chemical structure are noticeable. A comparative study found that the half-life of naphthenic mineral oil (~225 days) was much higher than castor oil (12 days) and two other synthetic bio-lubricants (~26 & ~20 days).[4] Equipped with an array of sustainable and renewable base oil structures, the growth of bio-lubricants, therefore, relies on efficient and low-cost production methods and superior tribological and anti-wear performance in a wide range of conditions. Although, notable hurdles lie within a bio-lubricant’s inherently poor oxidative stability, which can transform the base oil into a plastic-like consistency and high pour point.[1, 5] The presence of unsaturated bonds remains an ample site for unwanted reactions to occur, such as oxidation.[6] However, recent additive research efforts point towards a new direction that can help overcome these hurdles.
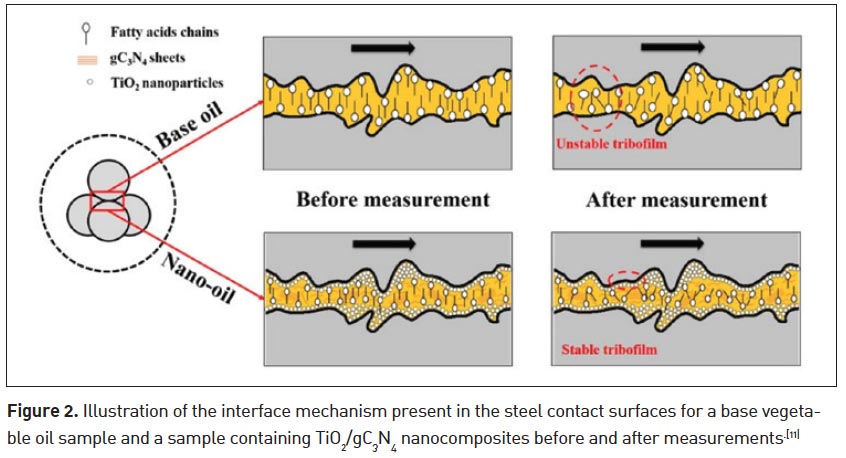
For EALs to become more mainstream, the means of production or synthesis, the source of raw materials, and cost are among the many non-physical variables which must be evaluated. Figure 1 depicts numerous chemical conversion routes in converting vegetable or waste cooking oil (WCOs) into a bio-lubricant.[7] For each conversion route, parameters such as the type of catalyst, catalyst dosage, temperature, molar ratios, and reaction times affect the efficiency of the conversion.
The study conducted by Zein et al [7] represents a comprehensive analysis of the production of dioleoyl ethylene glycol ester from fatty acid methyl esters (FAMEs) of WCOs, which considers each of these parameters to optimize bio-lubricant output. In terms of cost, precise comparisons between mineral and vegetable oils remain vague. However, the U.S. Environmental Protection Agency (EPA) hints that the higher base oil and additive costs result in bio-lubricants costing ~20% more than conventional products.[8] As additive research continues to develop, and environmentally-friendly legislation is pushed to incentivize green lubrication, the cost of implementing bio-lubricants will soon become favorable for industrial applications. [1]
Use of additives
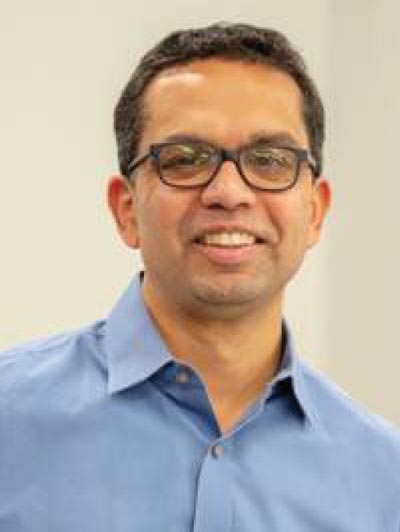
The enhancement of tribological performance and the range of applicable conditions is primarily accomplished through the use of additives. Recent additive research intersects the field of nanotechnology and tribology by pairing certain nanoparticles which act as effective solid lubricants with fluid or semi-fluid lubricants/greases. This process, which involves the stabilization and dispersion of nano additives such as multi-walled carbon nanotubes (MWCNs) or graphene, has represented an interesting method of directly bolstering bio-lubricant properties. For example, Rajaganapathy et al[9] found a reduction of the coefficient of friction (COF) and overall wear using palm and brassica oil upon additions of copper oxide (CuO) and titanium dioxide (TiO2) at various concentrations. An increase in thermal conductivity and viscosity of the “nano bio-lubricants” were also revealed for optimal nanoparticle weight percentages.[9]
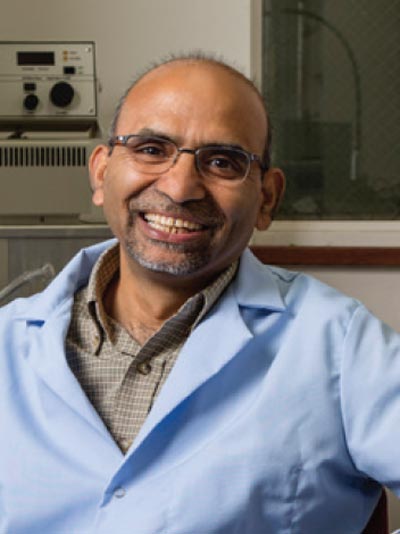
MWCNs have also particularly shown lubrication enhancements as tamanu oil exhibited shear-thinning behavior.[10] Ranjan et al[11] performed a series of tests using groundnut oil with the addition of a TiO2/gC3N4 nano additive. Figure 2 represents the proposed mechanism by which the nanocomposite affects the lubrication between the steel contact surfaces. In particular, TiO2/gC3N4 provided a mending effect by filling up wear volume after contact and by providing a smooth surface.[11] Nano additives serve as an effective tool in enhancing tribological properties. Most importantly, Ranjan et al[11] further realized from IR spectroscopic analysis that the addition of the nanocomposite prevented the oxidation of the base oil, which was attributed to the presence of nitrides and oxides protecting the unsaturated bond sites.[11] An enhancement in thermo oxidative stability was also discovered using a CuO nano additive dispersed within a chemically modified custard apple seed oil.[12]
Polymer additives
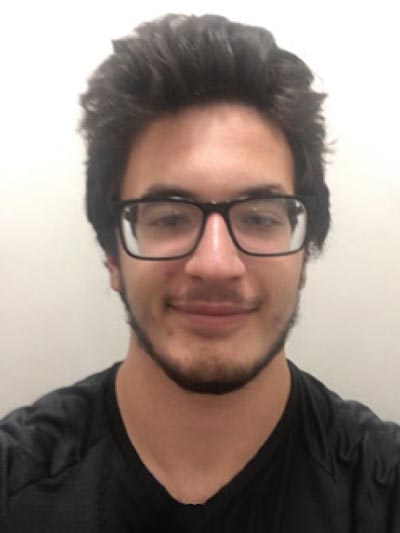
Polymer additives also present a similar opportunity in enhancing the mechanical properties of bio-lubricants even for more non-traditional applications. For instance, Feng et al[13] focused on applying bio-lubricants in artificial joints with the assistance of polystyrene nanospheres. Polystyrene was chemically grafted with poly (3-sulfopropyl methacrylate potassium salt) (PSPMA) through subsurface-initiated atom transfer radical polymerization (sSI-ATRP) to form PS-PSPMA. Using water as a lubricant, the polymer additive formed a thick hydration layer which greatly reduced the coefficient of friction from 0.19 to 0.06 and wear volume under variable loads (~10-50 N).[13]
The tribological performance of a polyol ester oil-based bio-lubricant has likewise demonstrated improvements with the addition of 2,2,6,6-tetramethylpiperidine-1-oxyl (TEMPO)-oxidized bacterial cellulose (NDCt) and a sorbitan monostearate (Span 60) surfactant to form N2S4.[14] COF and wear rate decreased by 79% and 49%, respectively, while thermal conductivity increased by 4% when compared to the ester-based control.[14]
In both polymer and nanoparticle additions, characterization of the mechanisms by which they impact lubrication or physical properties provides a deep understanding of the underlying processes. The impact of these additives on environmental sustainability must also be evaluated as phosphorus, carbon, and nitrogen can convert into oxides and form toxic products or blockage of filters.[11, 15] Nonetheless, the advent of additive research has paved a promising future for the growing application of environmentally acceptable lubricants and more renewable, biodegradable, and sustainable lubrication altogether.
Authors’ note: Mention of trade names or commercial products in this article is solely for the purpose of providing specific information and does not imply recommendation or endorsement by the U.S. Department of Agriculture. USDA is an equal opportunity provider and employer.
1 Koehler Instrument Company, 2 United States Department of Agriculture, Agricultural Research Service Eastern Regional Research Center
REFERENCES
[1] Shah, Raj, Woydt, Mathias and Zhang, Stanley. (2021). The economic and environmental significance of sustainable lubricants. Lubricants, 9. 21. 10.3390/lubricants9020021.
[2] Battersby, N. (2000). The biodegradability and microbial toxicity testing of lubricants – Some recommendations. Chemosphere, 41, 1011-27.10.1016/S0045-6535(99)00517-2.
[3] Pettersson, Anders. (2007). High-performance base fluids for environmentally adapted lubricants. Tribology International, 40, 638-645. 10.1016/j.triboint.2005.11.016.
[4] Luna, F. M. T., Cavalcante, J. B., Silva, F. O. N., & Cavalcante, C. L. (2015). Studies on biodegradability of bio-based lubricants. Tribology International, 92, 301-306. doi:10.1016/j.triboint.2015.07.007.
[5] Nosonovsky, M. and Bhushan, B. (2010). Green tribology: principles, research areas and challenges. Philosophical Transactions of the Royal Society A: Mathematical, Physical and Engineering Sciences, 368 (1929), 4677–4694. doi:10.1098/rsta.2010.0200 [a] Bayat, Reza & Lehtovaara, Arto. (2020). Friction and temperature mapping of environmentally acceptable gear oils. Tribologia: Finnish Journal of Tribology, 37, 4-12. 10.30678/fjt.96048.
[6] Kumar, Ajay, Thakre, Gananath, Arya, Pankaj, and Jain, Amar. (2017). Influence of operating parameters on the tribological performance of oleic acid-functionalized Cu nanofluids. Industrial & Engineering Chemistry Research, 56 (13), 3527-3541. 10.1021/acs. iecr.6b04375.
[7] Zein, Reda, Attia, N., Fouad, Mai, and El Sheltawy, Shakinaz. (2021). Experimental investigation and process simulation of biolubricant production from waste cooking oil. Biomass and Bioenergy, 144, 105850. 10.1016/j.biombioe.2020.105850.
[8] Environmental Protection Agency. Environmentally acceptable lubricants; Environmental Protection Agency: Washington, DC, USA. Available online: https://www3.epa.gov/npdes/pubs/vgp_environmentally_acceptable_lubricants.pdf (accessed on 20 June 2021).
[9] Rajaganapathy, C., Vasudevan, D., and Murugapoopathi, S. (2021). Tribological and rheological properties of palm and brassica oil with inclusion of CuO and TiO2 additives, Materials Today: Proceedings, 37 (2), 207-213. https://doi.org/10.1016/j.matpr.2020.05.032.
[10] Galgat, Sagar, Sharma, Ankit, Kumar, Gautam, Pudotha, Pramod, Gupta, Prince, Chowdary, Krishna, and Kotia, Ankit. (2021). Rheological analysis on tamanu oil for sustainable biolubricant in electric vehicle application. Int J Petrochem Sci Eng, 6 (1), 1-3. Available online: https://medcraveonline.com/IPCSE/IPCSE-06-00125.pdf
[11] Ranjan, N., Shende, R.C., Kamaraj, M., and Ramaprabhu, S. (2021). Utilization of TiO2/gC3N4 nano-additive to boost oxidative properties of vegetable oil for tribological application. Friction, 9, 273–287. https://doi.org/10.1007/s40544-019-0336-9.
[12] Pillai, Leelamani & Kumar, Gajendra. (2020). Investigation of tribological properties of chemically modified custard apple seed oil biolubricant dispersed with nano copper oxide. Journal of Shanghai Jiatong University, 16 (11), 272-284. Available online: https://shjtdxxb-e.cn/wp-content/uploads/2020/11/JSJ.U-2838.29-F.pdf
[13] Feng, H., Ma, Z., Zhang, Y., Liu, F., Ma, S., Zhang, R., Cai, M., Yu, B., and Zhou, F. (2020). Polystyrene nanospheres modified with a hydrophilic polymer brush through subsurface-initiated atom transfer radical polymerization as biolubricating additive. Macromolecular Materials and Engineering, 305 (6), 2000135. doi:10.1002/mame.202000135.
[14] Rahmadiawan, Dieter, Abral, Hairul, Nasruddin, Nasruddin, & Fuadi, Zahrul. (2021). Stability, viscosity, and tribology properties of polyol ester oil-based biolubricant filled with tempo-oxidized bacterial cellulose nanofiber. International Journal of Polymer Science, 2021, 5536047, 1-9. 10.1155/2021/5536047. https://www.hindawi.com/journals/ijps/2021/5536047/
[15] Rokosz, M.J., Chen, A.E., Lowe-Ma, C.K., Kucherov, A., Benson, D., Peck, M.C., and McCabe, R.W. (2001). Characterization of phosphorus-poisoned automotive exhaust catalysts. Applied Catalysis B: Environmental, 33, 205-215. 10.1016/S0926-3373 (01) 00165-5.